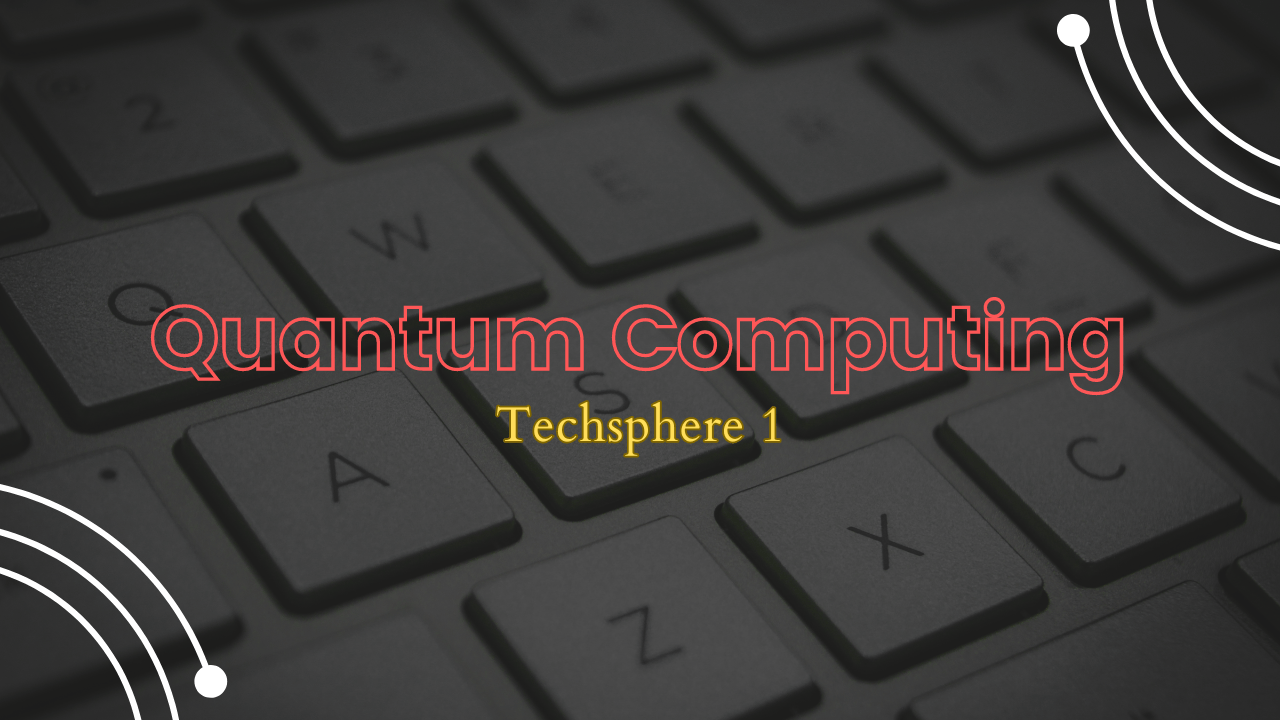
Quantum computing:
Quantum computing represents a revolutionary shift in processing power, offering the potential to solve complex problems that are currently unsolvable for classical computers. At its core, quantum computing manipulates quantum bits, or qubits, to perform operations. These qubits can exist in a state of superposition, enabling instantaneous correlation between qubits regardless of their physical separation. Utilizing the concepts of quantum mechanics, quantum computing is a cutting-edge approach in the field of cutting-edge technology that processes information in ways that conventional computers are unable to. In order to understand quantum computing, let us examine the basic ideas that underlie it:
Table of Contents:
1. Quantum Bits (Qubits): Exploring the latest buzz in quantum computing
Quantum bits, or qubits, are the fundamental components of quantum computing. Unlike classical bits that can exist in one of two states (0 or 1), qubits can exist in multiple states simultaneously, thanks to the principle of superposition. This unique property enables quantum computers to perform complex calculations in parallel. In the fascinating realm of quantum computing, quantum bits, or qubits, stand as the elemental building blocks that propel computation into the realm of quantum mechanics. Quantum supremacy denotes the point at which a quantum computer outperforms the most advanced classical computers in a specific task. Here are some types of qubits:
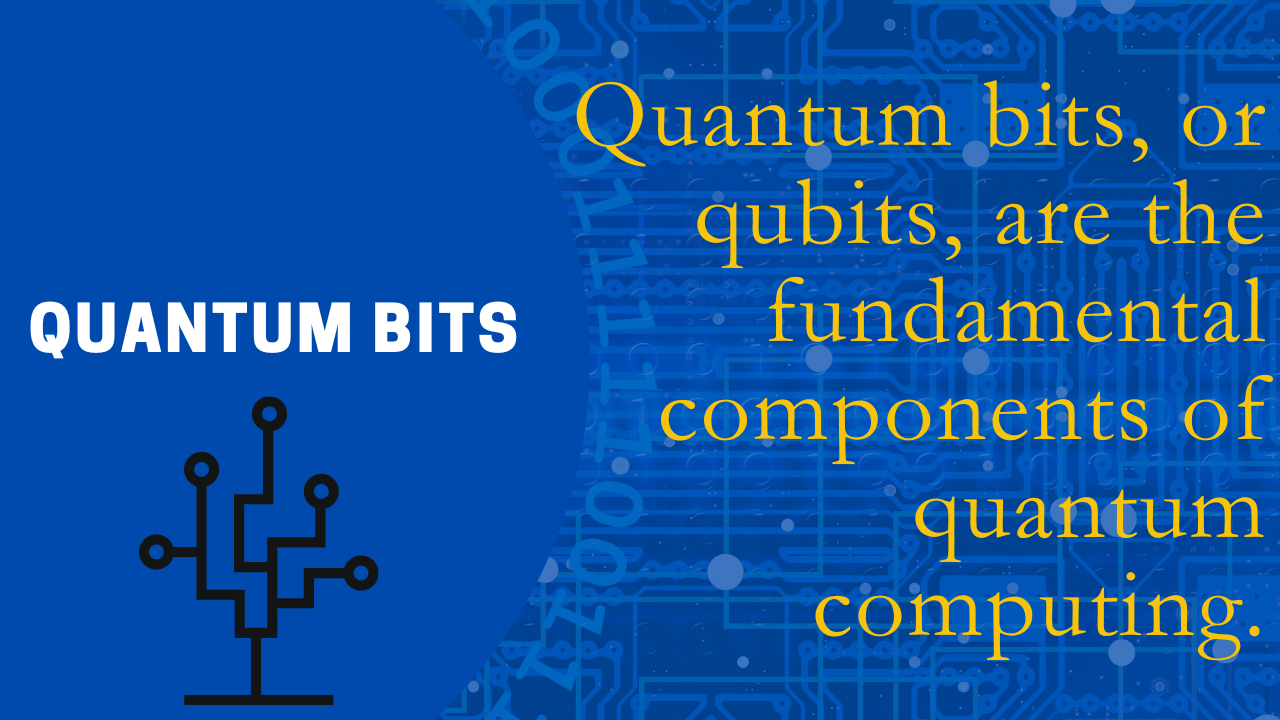
Types of qubits:
There are several physical implementations of qubits, each with its advantages and challenges:
Electron spin qubits: Electron spin qubits utilize the spin of electrons trapped in semiconductor materials to represent quantum information. They offer long coherence times and scalability, making them promising candidates for quantum computing.
Superconducting Qubits: Superconducting qubits are fabricated from superconducting materials and manipulated using microwave pulses. They are highly controllable and have been used to demonstrate various quantum algorithms and operations.
Trapped ion qubits: Trapped-ion qubits involve using ions confined in electromagnetic traps to store and manipulate quantum information. They offer high-fidelity quantum operations and have been employed in quantum simulation and computation experiments.
2. Superposition:
Superposition allows qubits to exist in a combination of 0 and 1 states simultaneously. This enables quantum computers to process a multitude of possibilities at once, vastly increasing their computational power compared to classical computers that handle one possibility at a time. In the realm of quantum computing, multitasking takes on an entirely new dimension. Quantum computers leverage a unique property called quantum parallelism, enabling them to perform multiple computations simultaneously. While quantum parallelism is a powerful concept, it’s essential to note that not all problems benefit equally from this approach. Let us explore the nature of superposition and how it affects the field of quantum computing:

3. Entanglement: Quantum Connectivity
Entanglement is a phenomenon where qubits become interconnected, and the state of one qubit is directly linked to the state of another, regardless of the physical distance between them. This interconnectedness allows quantum computers to perform operations collectively, enhancing their processing capabilities. In the enthralling landscape of quantum mechanics, the concept of quantum connectivity, often exemplified by entanglement, stands as a mysterious and profound phenomenon. While entanglement holds immense promise, creating and maintaining entangled states over long distances presents challenges. Following are some key features of entanglement:

Key Features of Entanglement:
- Non-locality: Entanglement violates the principle of locality, suggesting that the state of one particle can influence the state of another instantaneously, regardless of the distance separating them.
- Connection without Causation: Entangled particles show correlations that traditional cause-and-effect connections are unable to account for. Instead, their states are determined collectively rather than individually.
- Quantum Coherence: Entanglement is closely related to the concept of quantum coherence, where particles exist in superposition states. The entangled particles share a joint quantum state, which remains coherent until measured or disturbed.
4. Quantum Gates: Manipulating Qubits
In quantum computing, quantum gates are the analogues of classical logic gates. They perform operations on qubits, like state flipping and entanglement creation, to manipulate them. To solve complicated problems, these gates are arranged in particular sequences to create quantum algorithms. Quantum gates, the fundamental components of quantum circuits, control the manipulation of qubits in the fascinating field of quantum computing. Manipulation of quantum matter is not without difficulties. Among their vulnerabilities are errors, decoherence, and noise in quantum systems. Here are some types of quantum computing:

Types of Quantum Gates:
There are several types of quantum gates, each serving a specific purpose in quantum computation:
- Pauli Gates: Including the X, Y, and Z gates, these gates perform rotations around the X, Y, and Z axes of the Bloch sphere, altering the state of qubits.
- Hadamard Gate: The Hadamard Gate creates superposition by transforming a basis state into a superposition state, enabling parallel computation.
- CNOT Gate (Controlled-NOT): The CNOT gate is a two-qubit gate that flips the second qubit (target) if the first qubit (control) is in state 1, demonstrating entanglement and enabling quantum entanglement-based operations.
- Phase Gates: Phase gates, such as the S and T gates, introduce phase shifts to qubits, altering their phase angles and enabling interference-based operations.
5. Quantum Interference: Enhancing Precision
Quantum interference occurs when the probability amplitudes of different quantum states combine either constructively or destructively. This phenomenon allows quantum computers to increase the probability of correct answers and decrease the probability of incorrect ones, enhancing computational precision. In the intricate landscape of quantum computing, enhancing precision is a delicate art achieved through the phenomenon of quantum interference. This quantum dance of probabilities and amplitudes plays a pivotal role in refining computations and elevating the accuracy of quantum algorithms.
Quantum interference’s significance:
Quantum interference is crucial for the design and implementation of quantum algorithms and quantum gates. By controlling the interference between quantum states, researchers can perform parallel computations, enhance computational efficiency, and solve complex problems that are intractable for classical computers.
Opportunities and Difficulties:
While quantum interference offers immense potential for quantum computing, it also poses challenges. Maintaining coherence and controlling interference in the presence of noise and decoherence remains a significant hurdle. Researchers are actively developing techniques to mitigate these challenges and improve the stability and reliability of quantum interference-based operations.

6. Quantum Parallelism: Solving Many Problems at Once
Quantum parallelism is a unique feature that enables quantum computers to simultaneously evaluate multiple solutions to a problem. This capability is particularly advantageous for solving complex problems in fields such as cryptography, optimization, and simulation. In the extraordinary realm of quantum computing, the concept of solving many problems at once takes center stage through the phenomenon known as quantum parallelism. Unlike classical computers, which tackle tasks sequentially, quantum computers harness the power of superposition to explore multiple solutions simultaneously.
Quantum Parallelism: An Understanding
In classical computing, operations are performed sequentially, one after another, limiting the speed and efficiency of computations. However, in quantum computing, quantum parallelism allows qubits, the basic units of quantum information, to exist in superposition states, representing multiple computational paths simultaneously.
How is quantum parallelism implemented?
Quantum parallelism leverages the ability of qubits to exist in a superposition of states, enabling the execution of multiple computational tasks in parallel. This means that quantum algorithms can explore a vast number of potential solutions simultaneously, rather than sequentially, leading to exponential speedups for certain types of problems.

7. Quantum Measurement: Extracting Information
To extract information from qubits, a quantum measurement is performed. However, unlike classical bits that always produce a definite outcome, the measurement of a qubit collapses its superposition, providing a probabilistic result. Quantum algorithms are designed to exploit this probabilistic nature to obtain meaningful information. Quantum information extraction, centered around the process of measurement, is a cornerstone of quantum computing. Researchers grapple with the intricacies of probabilistic outcomes, and the act of extracting information from qubits opens pathways to transformative advancements in computation and cryptography.
Comprehending Quantum Measurements:
In quantum mechanics, the act of measurement collapses the wavefunction of a quantum system, determining its state with respect to a particular observable. As a result of this collapse, the system assumes a definite state that corresponds to the measurement outcome, unlike quantum states that are probabilistic before measurement.
What is the process of quantum measurement?
Quantum measurement involves applying a measurement operation to a quantum system, such as a qubit or a collection of qubits. This operation has an effect on the quantum state of the system, leading it to collapse into one of its possible eigenstates. The system’s initial state determines the likelihood of each possible outcome.

8. Quantum Supremacy: Achieving Superiority
When a quantum computer surpasses the most sophisticated classical computers in a particular task, it is said to have achieved quantum supremacy. Google’s 2019 achievement of quantum supremacy, a significant turning point in the field, illustrated the potential of quantum computing. Reaching quantum superiority is a crucial first step toward realizing the full potential of quantum computing. The quantum frontier has the potential to completely change how we solve complicated problems as researchers overcome challenges, enhance algorithms, and look into practical applications.
Future Directions and Challenges:
Although promising, attaining quantum supremacy presents a number of obstacles:
Error Correction: Quantum systems are susceptible to errors caused by noise, decoherence, and imperfect operations. Overcoming these challenges and implementing error correction techniques are crucial for realizing the full potential of quantum supremacy.
Scalability: Demonstrating quantum supremacy on larger, more complex problems requires scaling up quantum hardware while maintaining coherence and control over qubits. Advancements in qubit technology and system architecture are necessary to address scalability challenges.
Verification and Validation: Validating the results of quantum supremacy experiments and ensuring their reproducibility are essential for establishing credibility within the scientific community. Developing reliable methods for verifying quantum computations and benchmarking quantum systems is critical for advancing the field.

Conclusion:
To sum up, quantum computing, which is based on the ideas of quantum mechanics, is a revolution in the field of computing. Its capacity to take advantage of quantum parallelism, entanglement, and superposition opens the door to solving challenging issues that were previously unsolvable for conventional computers. We expect extraordinary breakthroughs that will completely change the way that information is processed, and problems are solved, as long as research and development on quantum computing continue.
FAQS:
Q1. What is quantum computing?
Ans. Quantum computing presents a promising solution to complex puzzles that are currently beyond the capabilities of traditional computers due to its revolutionary approach to processing power. Fundamentally, quantum computing works by manipulating quantum bits, or qubits, to carry out operations.
Q2. How can I learn about quantum computing?
Ans. There are a few ways you can learn about quantum computers. You can read about them in books or articles; you can also attend quantum computing conferences; or you can take online classes.
Q3. How does quantum computing differ from classical computers?
Ans. It differs from classical computers in the way the information is represented and processed.
Q4. What is a quantum hardware system?
Ans. A quantum hardware system is about the size of a car, made up mostly of cooling systems to keep the superconductor processor at its ultra-cold operational temperature.
Q5. What is the basic unit of information in quantum computing?
Ans. The basic unit of information in quantum computing is the qubit, similar to the bit in traditional digital electronics.